Stellar Structure and Evolution
Stars are the source of almost all of the light our eyes see in the sky. Nuclear fusion is what makes a star what it is: the creation of new atomic nuclei within the star’s core. Many of stars’ properties — how long they live, what color they appear, how they die — are largely determined by how massive they are. The study of stellar structure and evolution is dedicated to understanding how stars change over their lifetimes, including the processes that shape them on the inside.
Our Work
Center for Astrophysics | Harvard & Smithsonian researchers study stellar structure and evolution in many ways:
-
Studying fluctuations in light on nearby stars to determine their internal processes. While most stars appear too small to distinguish surface features, astronomers can infer variations in their interiors by how their light fluctuates. Those changes are due to “starspots” — dark spots created by magnetic variations in a star — and starquakes. For example, astronomers recently discovered that Proxima Centauri, the nearest star to the Sun, has starspots. That discovery was surprising, because researchers previously thought red dwarf stars like Proxima Centauri don’t have strong magnetic fluctuations.
Proxima Centauri Might Be More Sunlike Than We Thought -
Monitoring sound waves running through the interiors of Sun-like stars. These starquakes produce variations in the star’s light. Much like earthquakes provide hints about Earth’s, these sound waves allow astronomers to measure what’s going on inside stars. Using NASA’s Kepler observatory and other telescopes monitoring stars for exoplanet signals, researchers measure the fluctuations of light caused by starquakes.
Solar-Like Oscillations in Other Stars -
Studying stars that are similar to the Sun at other stages in evolution. We can only observe our Sun at this particular time of its life, but astronomers can see its past and future by looking at similar stars earlier or later in their cycle. Astronomers observe newly born Sun-like stars to determine what ours may have been like, and the effect that had on planet formation.
Young Sun-like Star Shows a Magnetic Field Was Critical for Life on the Early Earth -
Observing stars in the final stages of their lives. These giant stars pulsate and shed huge amounts of matter. Studying them reveals how they enrich interstellar space with new atoms, and how pulsation relates to physical processes deep in the star’s interior. Using the National Radio Astronomy Observatory’s Atacama Large Millimeter/submillimeter Array (ALMA) and other observatories, astronomers can identify the composition of the “winds” from aging stars.
Pulsation-Driven Winds in Giant Stars -
Identifying stars at all stages of life — including places where both dying and newborn stars coexist. Using NASA’s Chandra X-ray Observatory and other telescopes, astronomers have learned that the violent final stages of a star’s life can spur the creation of new stars, by compressing interstellar gas until it collapses under its own gravity to make protostars. In other instances, X-ray light from a binary system with a black hole or neutron star illuminates a star-forming region, which is opaque to visible light, but transparent to X-rays.
A Stellar Circle of Life -
Measuring the ages of stars to understand how they change over the course of their lives. Stars begin their lives spinning fast, and slow down gradually over time. Researchers want to know exactly how that rate changes, and how it reflects the aging of the star itself. Using NASA’s Kepler observatory and other instruments, astronomers have tracked starspots to measure the spinning of stars in a single cluster.
Stars' Spins Reveal Their Ages -
Studying YSOs and their environments, as a way to determine how stars have the masses they do. The mass of a star dictates its life cycle, and that mass is set during its growth period before it’s even a star. Using the CfA’s Submillimeter Array (SMA) and other telescopes capable of seeing through the gas and dust around newborn stars, astronomers can track the evolution from protostar to star.
SMA Unveils How Small Cosmic Seeds Grow Into Big Stars
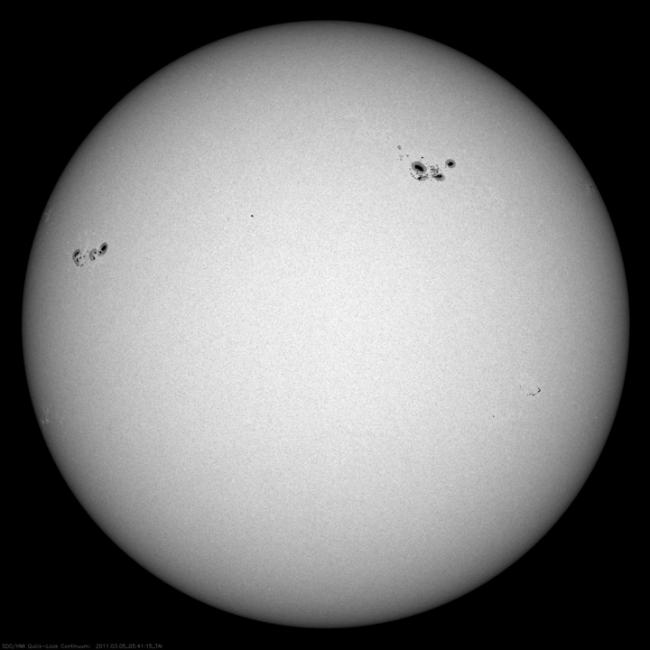
This NASA's Solar Dynamics Observatory image reveals two large sunspot groups on the surface of the Sun. Sunspots and starspots are produced by magnetic activity, providing information about the internal structure of stars.
A Star Is Born
All stars begin their lives in dense interstellar clouds of gas and dust. Even before they become stars, though, much of their future life and structure is determined by the way they form.
A star is defined by nuclear fusion in its core. Before fusion begins, an object that will become a star is known as a young stellar object (YSO), and it passes through two major stages of development.
-
During the protostar phase, the YSO is still gathering mass onto itself in the form of gas and dust. Protostars are completely hidden in visible light, so all the information we have about them comes from infrared, submillimeter, and X-ray observations. The protostar’s gravity gathers mass into a spinning circumstellar disk, and some of the matter is funneled into powerful jets shooting away from the YSO. These processes help determine the mass of the eventual star, and as such dictate much of the rest of the star’s life.
-
During the pre-main-sequence (PMS) phase, the YSO contracts and heats up. New planets form out of the remains of the circumstellar disk. The specific way the YSO behaves depends on how much mass it gathers. Lower mass stars like the Sun pass through a stage of wild fluctuations as they lose their shrouds of gas and dust, during which they are called “T Tauri stars”. Higher mass PMS stars produce huge amounts of radiation, which can drive the surrounding gas away. This can throttle the formation of other stars, either preventing them from forming or keeping them at lower masses.
The jets and outflows of particles from YSOs can have a profound influence on the surrounding nebula. Since many stars form in a cluster from the same pool of gas and dust, they affect each other’s growth and development in profound ways.
All About Mass
Once YSOs have contracted and heated enough, fusion of hydrogen into helium begins in their cores and they become main sequence stars. The rate of that fusion increases with the mass of the star, so the most massive stars are the shortest-lived.
The lowest-mass stars are known as red dwarfs or M dwarfs. These experience convection — the circulation of matter — throughout their interior. That means they burn for a very long time, giving them lifetimes much longer than the 13.8 billion years the universe has been around. None of these stars have lived through their entire lifecycle yet.
The Sun is a moderate mass star with a lifetime of roughly 10 billion years; we’re currently about halfway through the Sun’s main sequence. Stars in this middle range of mass have a distinct core where fusion takes place, and that limits the available supply of hydrogen to fuse into helium. Once that supply is exhausted, the star leaves the main sequence and swells into a red giant. The core then collapses slightly as it begins fusing helium into carbon and oxygen. Once the available helium supply is used up, the star sheds its outer layers, exposing the remnant of its core. This remnant is a white dwarf.
The highest mass stars consume their available hydrogen even more quickly, passing through the main sequence and helium-fusion phase in a much shorter amount of time. However, these stars have enough mass to keep fusion going, producing heavier elements up to iron. Elements beyond iron on the periodic table require more energy to fuse than is released by the fusion process, so the core of these stars can’t keep up the work. The core collapses under gravity, and the outer layers of the star are blown off in a supernova explosion. For the most massive stars, the cores collapse into black holes; the slightly less massive stars leave behind neutron stars.
Aging Stars
During the post-main-sequence evolution when stars grow huge, they may also pulsate in and out due to instabilities in the outer layers of the stellar envelope. These pulsating stars include the Cepheid variables, used in measuring distances within the Milky Way and to nearby galaxies.
In addition, massive stars in the last stages of life are the source of new elements. Fusion during the giant phases of stellar evolution produces elements like carbon, oxygen, and silicon that may be cycled toward the outer layers of the star. For the most massive stars, neutrons from fusion bombard atoms in the star to make yet more elements, including technetium, a rapidly-decaying element that doesn’t exist naturally on Earth. The more stable atoms from the dying star appear in the spectrum of its light, and are shed into interstellar space as the star dies.
The Seismology of Stars
We can’t see directly into a star’s interior. However, just as earthquakes on Earth’s surface reveal what’s going on inside the planet, the behavior of material on the surface of stars provides researchers with information about the interior. Asteroseismology is the study of vibrations of a star.
Naturally, the Sun is the star easiest to study. Researchers have measured the patterns of waves on the surface set up by the flow of atoms and energy deep inside the Sun. For more distant stars, astronomers observe variations in light from these processes. In some stars, the churn of hot matter is enough to produce “starquakes”: more violent fluctuations in the star’s behavior.
- Solar & Heliospheric Physics
- Stellar Astronomy
- The Energetic Universe
- The Milky Way Galaxy
- Theoretical Astrophysics
- Computational Astrophysics
Related News
Astronomers Discover New Building Blocks of Complex Organic Matter
CfA Celebrates 25 Years with the Chandra X-ray Observatory
Extreme Eruption on Young Sun-like Star Signals Savage Environment for Developing Exoplanets
Extreme Weight Loss: Star Sheds Unexpected Amounts of Mass Just Before Going Supernova
Stellar Surf's Up: Monster Waves as Tall as Three Suns are Crashing upon a Colossal Star
Streamlining the Search for Black Holes
'Black Hole Police' Discover a Dormant Black Hole Outside of the Milky Way Galaxy
Scientists Explain Mysterious Finger-like Features in Solar Flares
Spacecraft Enters the Sun's Corona for the First Time in History
Astronomers Observe a New Type of Binary Star Long Predicted to Exist
Projects
AstroAI
GMACS
For Scientists
Sensing the Dynamic Universe
SDU Website
Telescopes and Instruments
1.5-meter Tillinghast (60-inch) Telescope
CfA Operated (OIR) | Open to CfA Scientists | Active
Visit the 1.5 Meter (60 Inch) Tillinghast Telescope Website
Arcus
See Arcus Website
Hungarian-made Automated Telescope Network (HATNet)
Visit the HATNet Website
Kepler/K2
Visit the Kepler/K2 Website
MEarth
Visit the MEarth Website
MINiature Exoplanet Radial Velocity Array (MINERVA)
Visit the MINERVA Website
Transiting Exoplanet Survey Satellite (TESS)
Visit the TESS Website